Mars is famous as the Red Planet for its rusty iron-rich dust. Although it may be a cold desert today, it once hosted some of the largest volcanoes in the solar system. In the hunt for space mining opportunities, Mars’s fiery past might hold the key to valuable mineral deposits. On Earth, volcanic activity often concentrates useful metals and minerals. Did the same happen on Mars?
By examining how Martian volcanoes worked and how they resemble (or differ from) Earth’s, we can start to predict what mineral bounty might await future Mars explorers. Let’s dive into the planet’s ancient history and see what clues it offers about valuable minerals beneath the surface.
Mars and Its Mighty Volcanoes: A Planet Forged by Fire
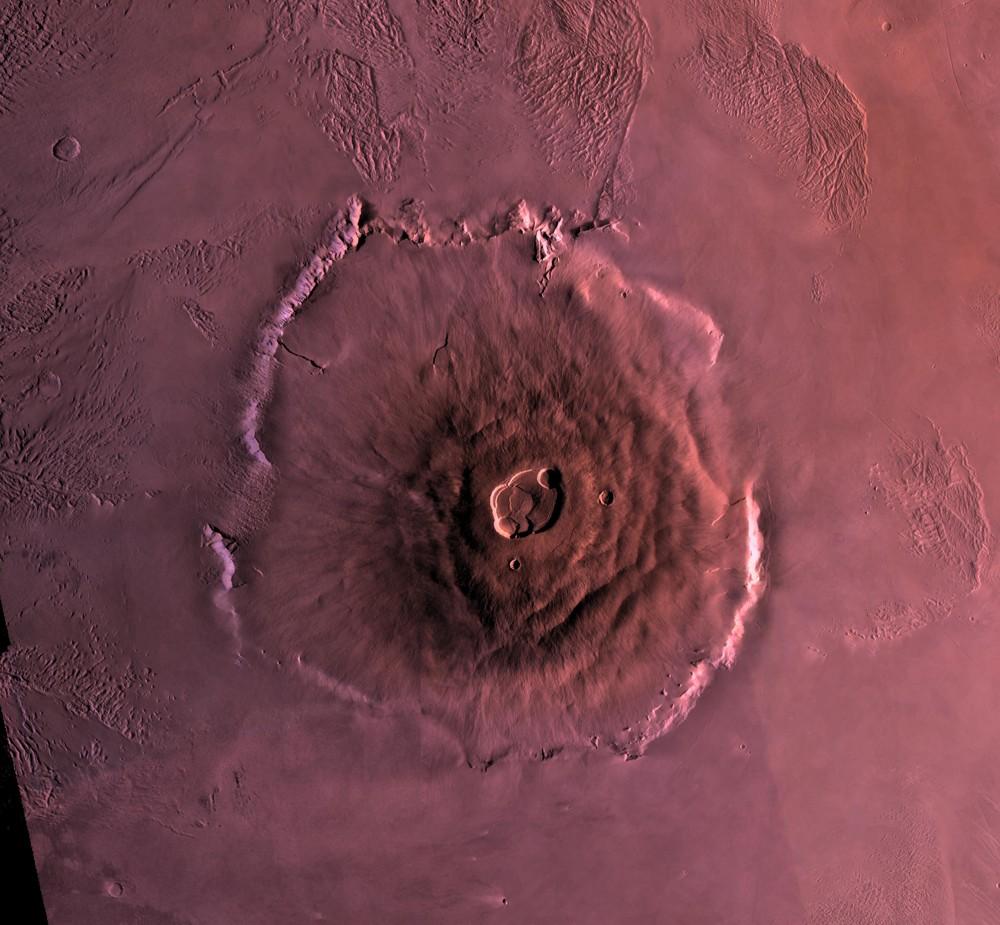
Mars was once a volcanically active world. Billions of years ago, its interior heat fueled massive volcanoes that dwarfed those on Earth. The prime example is Olympus Mons, a giant shield volcano about 21–27 kilometers tall and over 600 km wide. That’s roughly three times the height of Mount Everest and about the size of Arizona in area. Mars hosts a whole province of huge volcanoes in regions like Tharsis (home to Olympus Mons and three others) and Elysium.
These volcanoes could grow so large because Mars lacks the plate tectonics that Earth has. On Earth, moving tectonic plates carry volcanoes off their hotspots, limiting their size, but Mars’s crust stayed stationary over hot spots, allowing magma to pile up in one location for eons. The result was hundreds of millions of years of eruptions building enormous volcanic edifices.
Martian volcanism was most intense in the distant past (the Noachian and Hesperian epochs, over 3 billion years ago), but continued into more recent geological times. Some lava flows on Mars are relatively young – there’s evidence of eruptions a few hundred million years ago, and possibly even minor activity in the last 50 million years. Today, Mars appears geologically quiet on the surface; no active eruptions have been observed by our spacecraft.
Nonetheless, the towering volcanoes and vast hardened lava plains are a testament to a fiery past that did more than reshape the landscape: it may have redistributed and concentrated minerals in the Martian crust.
Shaped by Basalt
Most Martian lava is basaltic, a dark volcanic rock rich in iron and magnesium. When you see Mars’s grey-black volcanic plains or the dark dunes of basaltic sand, you’re looking at solidified basalt lava. The dark sand dunes common on Mars owe their color to volcanic basalt and contain minerals like magnetite, chromite, and ilmenite (iron- and chromium-bearing oxides) derived from those lavas (known from both surface observations and Martian meteorites).
Wind has helpfully gathered these heavy mineral sands into concentrated dunes, which could provide iron, titanium, and chromium for construction and manufacturing. In essence, Mars’s basaltic volcanoes paved the planet with raw material. Ore deposits often form with the help of heat, and volcanoes are tremendous heat engines. Over time, these massive volcanoes may have enabled two key mineral-forming processes:
- Magmatic differentiation (separation of heavy minerals during magma cooling),
- Hydrothermal activity (metal-rich fluids depositing ores in cracks and fractures).
How Volcanoes Can Concentrate Minerals: Earth Analogs
Why would volcanic activity create valuable mineral deposits? The answer lies in some basic geology that applies to both Earth and Mars.
A. Magmatic Differentiation (Crystal Settling)
As magma cools, heavy minerals like chromite, magnetite, and sulfides sink and settle to the bottom of the chamber due to gravity, forming layered intrusions of dense, metallic elements. Earth’s Bushveld Complex (rich in platinum) and Norilsk (a major nickel-copper mine) are prime examples. If similar processes occurred in Martian magma chambers, Mars might host hidden pockets of valuable metals.
B. Hydrothermal Activity (Hot Water Circulation)
The second mechanism needs not just heat but also water. Volcanoes often occur in areas with groundwater or can create their own water by melting ice or releasing fluids. Hot magma heating groundwater = a hydrothermal system, essentially a natural hot spring or geothermal vent, creating systems that dissolve metals and later deposit them as ore veins (gold, silver, copper, etc.).
On Earth, this leads to massive deposits in volcanic belts like the Andes or the Pacific “Ring of Fire”. Mars also shows evidence of past hydrothermal activity, raising the possibility that similar metal-rich veins formed long ago.
Martian Minerals vs. Earth’s Volcanic Treasures
Earth’s volcanoes yield enormous mineral wealth. Could Mars offer analogs?
Deposit Type | Earth Example | Martian Evidence |
Hydrothermal veins (Au, Cu, Ag) | Andes, Nevada, Japan | Gypsum veins (Opportunity), silica hot springs (Spirit) |
Magmatic sulfides (Ni, Cu, PGE) | Norilsk, Bushveld Complex | Nickel/copper in meteorites; potential near large volcanoes |
Oxide-rich sands (Fe, Ti) | Hawaiian black sand beaches | Dunes with magnetite and ilmenite; easily collectable |
Sulfur/sulfates | Volcanic fumaroles, acid lakes | Widespread sulfate salts, sulfur-bearing minerals across Mars |
Silica deposits | Geyser basins, hot springs | High-purity opaline silica (Spirit), tridymite (Curiosity) |
Hydrothermal seafloor deposits | Mid-ocean ridges, Cyprus ophiolites | Eridania Basin’s thick hydrothermal sediment layers |
Terrestrial volcanoes leverage both these processes to create mineral wealth. Mars, as far as we know, had plenty of heat and volcanic activity in the past. By analogy, they could have formed similar deposits. The big question is: did it also have the water and the right conditions to do so? Let’s see what Mars rovers and orbiters have found.
What We Know So Far (What Mars Missions Have Found)
How do we know all this about Martian minerals? Robotic missions – orbiters, landers, and rovers – have thus far been our geologists on the ground (and in the sky). They have already made some “prospector-style” discoveries, even if mining wasn’t their primary goal. Here are some of the highlights:
Opportunity Rover (Meridiani Planum) – Hematite, Sulfates, and Meteorites
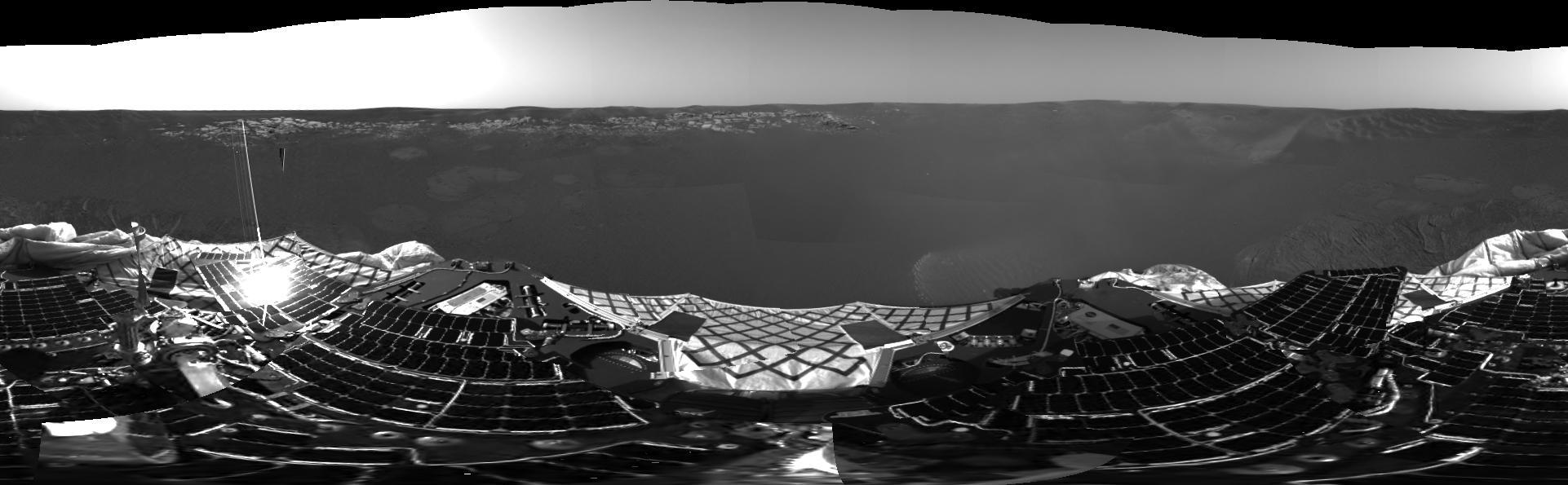
The Opportunity rover landed in 2004 in Meridiani Planum because a spectrometer from orbit saw signs of hematite there. On the ground, Opportunity discovered those hematite “blueberries” (tiny concretions that form in wet sediment), which were rich in iron (hematite). This was key evidence for past liquid water, but from a resources perspective, it also means free iron oxide pellets lying on the ground that could even be used to produce iron metal.
Opportunity also found veins of gypsum (calcium sulfate), which likely formed from mineral-rich water moving through a crack. What’s interesting is that this happened at the meeting point of sulfate-rich sandstone and older volcanic rocks at Endeavour Crater’s rim – essentially groundwater interacting with volcanic rock, which is a recipe for hydrothermal mineral formation.
Opportunity even stumbled upon metallic iron-nickel meteorites sitting on Mars’s surface. While not from Mars itself, those meteorites are rich in iron (one was 93% iron metal) and could also be resources – they required no mining at all, just simply scooping up! These discoveries show that Mars’s surface has accessible pockets of useful minerals even before we dig deep.
Spirit Rover (Gusev Crater) – Hot Spring Silica and Ancient Volcanoes
On the other side of Mars, the Spirit rover explored Gusev Crater (which was thought to be an old lakebed) and found layered rocks at a plateau called Home Plate, which turned out to be formed by volcanic explosions (likely a hydrovolcanic eruption where magma met water). Around Home Plate, Spirit made one of its most remarkable finds: patches of almost pure opaline silica (SiO₂·nH₂O). How do you get pure silica?
On Earth, either from acid vapors coming out of a volcano (fumaroles) or from a hot spring depositing silica. In either case, water is involved. The evidence suggested that Spirit had found the remnants of an ancient hot spring or fumarole on Mars – a strong sign of hydrothermal activity in a volcanic setting.
This was huge for science (as a possible habitat for ancient life), but it also means Mars did have the kind of geothermal systems that create mineral deposits. Along with silica, Spirit’s instruments detected other alteration minerals in Columbia Hills, like sulfates and iron oxides, all indicating that water + volcanic heat had altered the rocks.
Curiosity Rover (Gale Crater) – Layered Sediments and Varied Mineralogy
Although not a volcano, but a mound of sedimentary rock, at Gale Crater’s Mt. Sharp, Curiosity identified clay minerals and hematite in mudstone layers (clays often form by weathering of volcanic ash in water), and in higher layers it found lots of sulfate salts. The interesting twist was the discovery of that rare mineral tridymite in one rock sample. Its presence hinted that maybe a volcano somewhere was erupting ash that got into Gale Crater’s lake.
This implies not all Martian volcanism was basaltic; some might have been more like Earth’s cone volcanoes with evolved magma. If Mars had such volcanism, it would broaden the mineral prospects (e.g., more silica usually means potential for minerals like quartz veins, pegmatites with exotic elements, etc.).
Also, Curiosity drilled into Martian rocks and indirectly has shown that Martian rocks contain a mix of elements needed for life and useful to people (phosphorus, sulfur, chlorine, etc.).
Mars Reconnaissance Orbiter (MRO) – Scouting from Above
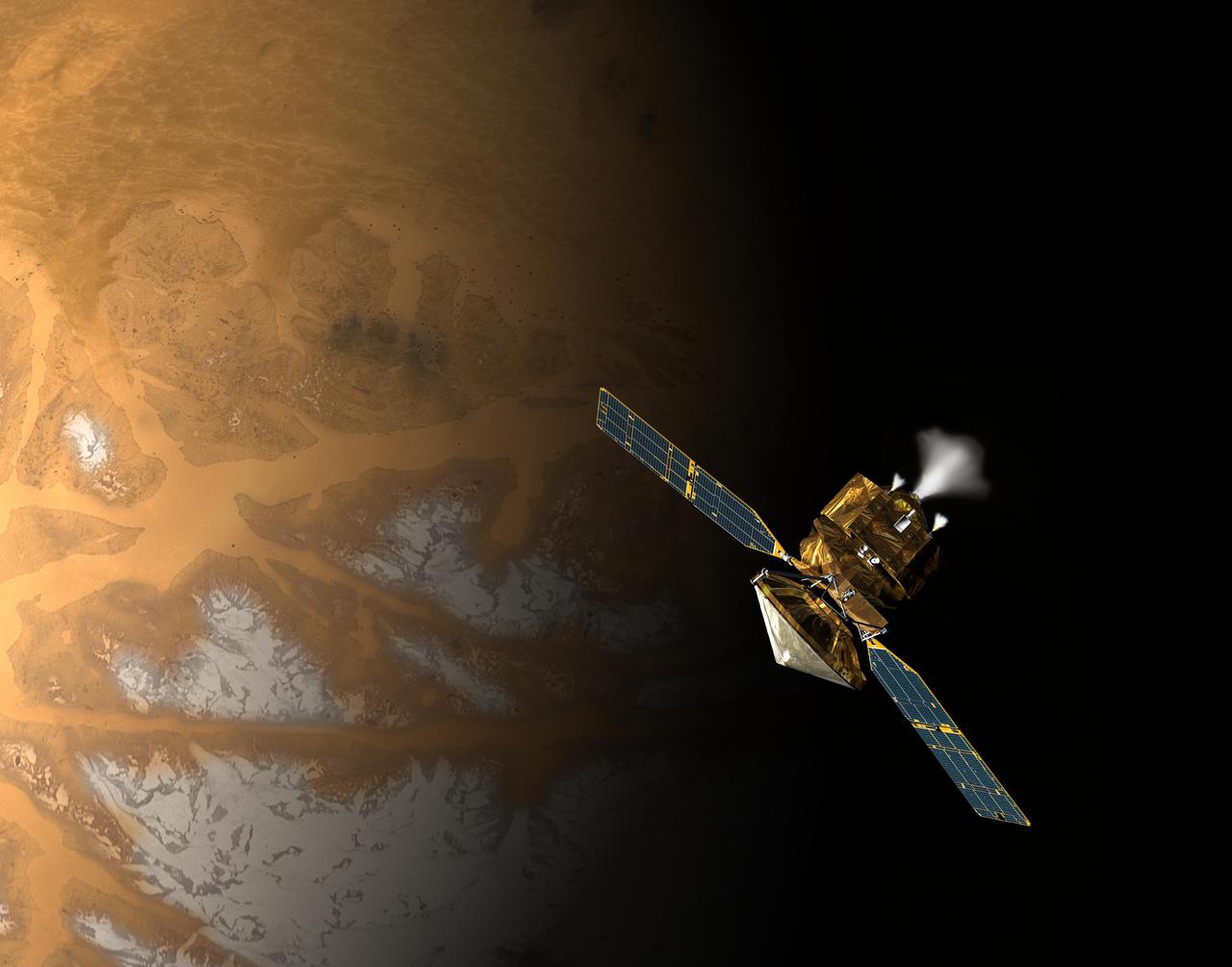
MRO’s instruments, like the CRISM spectrometer, have been instrumental in mapping minerals across Mars from orbit. CRISM has identified hundreds of locations with clay minerals, sulfates, silica, and other hydrated minerals on the Martian surface. Many of these are in ancient terrain, often around what appear to be ancient hydrothermal systems or water-rich environments.
One particularly relevant MRO finding was at Auki Crater: signs of minerals like smectite (clay), silica, zeolites, serpentine, carbonate, and chlorite were found in ridges inside this impact crater. This assemblage is characteristic of impact-induced hydrothermal systems on Earth.
While we haven’t detected rich metal ores at Auki, the mineral evidence of hydrothermal alteration is there.
Likewise, MRO found that in the giant Eridania basin (mentioned earlier), the mix of minerals points to seafloor hydrothermal deposition. MRO has essentially mapped out the most interesting mineral hotspots for future missions. We now know where the clays, sulfates, carbonates, iron oxides, etc., are concentrated on the surface.
The Million Dollar Question: Could Mars Be Mined?
While exporting metals to Earth is unlikely in the short term due to launch costs, Mars may become self-sufficient through local mining. Even without deep drilling, Mars offers some surface-accessible minerals:
- Hematite spheres and magnetite sands (iron),
- Ilmenite dunes (titanium),
- Gypsum veins (sulfur, construction materials),
- Silica-rich soils (potential for electronics and water extraction),
- Meteorites rich in metallic iron and nickel.
Space agencies and visionaries certainly think Mars will be an outpost for humanity in the future, which will rely on these materials to support in-situ resource utilization (ISRU). In the near term, that means things like digging up regolith (soil) to make bricks, extracting water from subsurface ice, and generating oxygen from the atmosphere or perchlorate salts.
Future Prospects
One advantage is that Mars’s gravity, while stronger than the Moon’s, is still only 38% of Earth’s. That makes it easier to excavate and haul materials (though still not trivial). But before any mining, we have to find the good ore first. The evidence suggests volcanic regions and ancient hydrothermal areas are prime targets. We’ve already identified some key regions of interest, for example:
- The flanks of big volcanoes like Olympus or Elysium Mons, where magma may have differentiated;
- The interiors and rims of large impact craters that show mineral alteration (could be ancient hydrothermal mines);
- Areas with exposed dikes and veins visible from orbit (potential pathways of ore-forming fluids).
What We Would Need to Make It Happen
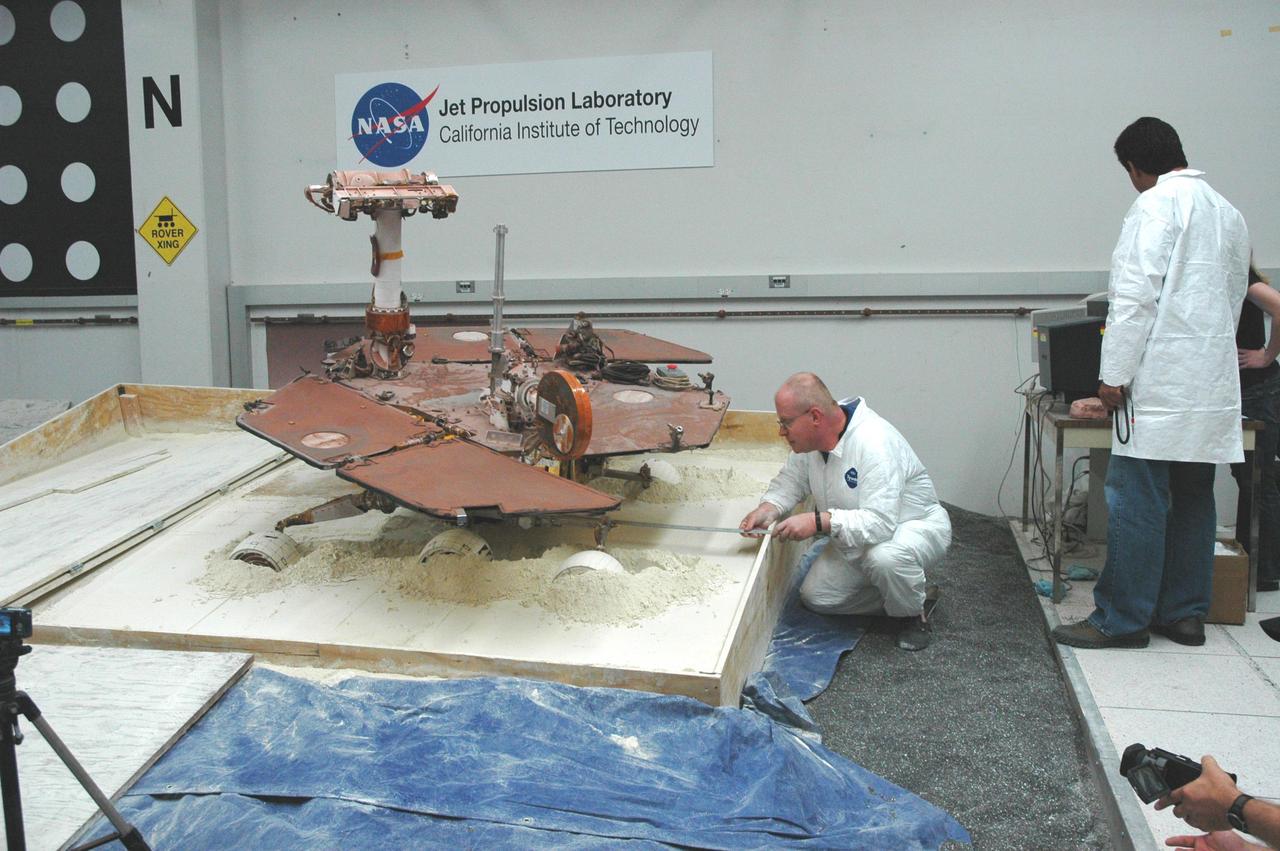
However, there are some key future developments we would need to be able to tap into these riches, such as:
Resource Mapping
Using advanced orbiters, AI-driven drones, or rover-mounted geophysical sensors to detect metal anomalies and dense rock formations. Scientists have suggested sending flying drones with magnetic and gravity sensors to skim the Martian surface to detect the subtle signals of dense metal deposits underground.
Mars’s thin atmosphere and wide-open plains would be suitable for lightweight autonomous aircraft (the Ingenuity helicopter, currently scouting for the Perseverance rover, has proven flight is possible on Mars). An aerial prospector could map variations in Mars’s gravity or magnetic field that indicate concentrations of heavy minerals or ores.
Autonomous Extraction
If promising deposits are found, the next step is extraction technology – developing low-gravity mining machines, magnetic separators, or solar-powered furnaces for local metallurgy. Mining on Mars would have challenges, such as the cold, the dust, the need for autonomous or remote-operated machinery (until lots of humans are present).
But it also has opportunities: for example, open-pit mining might be easier with lower gravity and no rain to worry about. Mars’s regolith is also not as weathered as Earth’s soil (no biological activity, etc.), so in many places, it’s essentially crushed rock ready to process. For instance, those magnetite-ilmenite dunes could be harvested with a simple front loader and run through a magnetic separator to pull out pure iron ore and titanium minerals.
Iron from Martian hematite or magnetite could be smelted (with the help of solar or nuclear power) into steel for building habitats. Chromium and titanium could make strong alloys for machinery that must handle Mars’s environment.
Volcanic Foothills and Dikes
For more precious metals like gold, platinum, or rare earths, it’s highly speculative whether Mars has any rich lodes of these. If they exist, they might be deep or in very specific geological settings (perhaps ancient hot spring deposits or the base of long-solidified magma chambers). Early Mars settlers will likely focus on the “low-hanging fruit” – abundant materials like iron, aluminum (from basalts and clays), sulfur (from sulfates), carbon (from CO₂ in the atmosphere or carbonates in rock), and, of course, water ice.
But as infrastructure grows, prospecting deeper will become feasible. One could envision a human mission in, say, Valles Marineris (the great canyon system) finding exposed mineral veins in the cliff walls, or a team setting up a mining camp near the foothills of a volcano where orbital surveys showed a strong metal anomaly.
Why Do We Care? Scientific and Economic Potential of Future Missions
From an economic standpoint, exporting minerals from Mars to Earth is unlikely to be viable in the foreseeable future, given the high cost of interplanetary transport. However, using Mars’s minerals on Mars will be absolutely vital if we are to have bases or colonies there. Every bit of steel or copper wire or silicon chip that can be manufactured on Mars from local materials is one less thing that has to be hauled from Earth.
In that sense, Mars’s volcanoes might one day provide the mines that support a Mars colony’s industry. Resources mined on Mars could also be used to build large spacecraft or satellites in Mars orbit or on Phobos (one of Mars’s moons), which might then be sent elsewhere. Such scenarios are distant, but they often start with the question: What does Mars have that we can use? And as we’ve seen, Mars has quite a lot – if we’re clever enough to extract it.
Over time, Martian volcanoes could evolve from landmarks of curiosity into vital infrastructure hubs for off-world industry. But beyond industrial use, exploring the Red Planet also means uncovering its history. Each mineral zone is a time capsule of Mars’s geology and resource potential, climate, and possible environments for past life. For example:
- Silica hot springs are potential astrobiological hotspots.
- Metal-rich hydrothermal veins could preserve biosignatures.
- Ancient mineral-rich basins may offer insight into Mars’s early atmosphere and tectonics.
By “mining” Mars, we will also be learning Mars, reading the records left in its rocks.
Forged in Fire, Waiting to Be Found
Mars’s volcanic past laid the foundation for a potentially resource-rich planet. From massive magma chambers to mineral-laden hydrothermal springs, Martian geology mirrors many ore-forming processes on Earth. Terrestrial volcanoes have given us rich deposits of metals and minerals, and many of the prerequisites for those processes existed on Mars as well.
We’ve already seen iron-rich sands, sulfur-bearing rocks, high-silica zones, and mineral veins, all telling us that valuable materials are present, even if the richest lodes remain undiscovered. What we lack so far is the “smoking gun” of, say, a gold-laden quartz vein sticking out of a Martian crater – but our exploration has really only scratched the surface (literally).
As Mars exploration accelerates, its volcanoes may transition from ancient relics to one day providing us with iron, titanium, and more, forged deep in the planet’s interior, waiting to be tapped in beneath the dust.